
Lichens are everywhere—grayish-green patches on tree bark on the Duke campus, rough orange crusts on desert rocks, even in the Antarctic tundra. They are “pioneer species,” often the first living things to return to barren, desolate places after an extreme disturbance like a lava flow. They can withstand extreme conditions and survive where nearly nothing else can. But what exactly are lichens, and why does Duke have 160,000 of them in little envelopes? I reached out to Dr. Jolanta Miadlikowska and Dr. Scott LaGreca, two lichen researchers at Duke, to learn more.

According to Miadlikowska, a senior researcher, lab manager, and lichenologist in the Lutzoni Lab (and one of the Instructors B for the Bio201 Gateway course) at Duke, lichens are “obligate symbiotic associations,” meaning they are composed of two or more organisms that need each other. All lichens represent a symbiotic relationship between a fungus (the “mycobiont”) and either an alga or a cyanobacterium or both (the “photobiont”). They aren’t just cohabiting; they rely on each other for survival. The mycobiont builds the thallus, which gives lichen its structure. The photobiont, on the other hand, isn’t visible—but it is important: it provides “food” for the lichen and can sometimes affect the lichen’s color. The name of a lichen species refers to its fungal partner, whereas the photobiont has its own name.

Unlike plants, fungi can’t perform photosynthesis, so they have to find other ways to feed themselves. Many fungi, like mushrooms and bread mold, are saprotrophs, meaning they get nutrients from organic matter in their environment. (The word “saprotroph” comes from Greek and literally means “rotten nourishment.”) But the fungi in lichens, Miadlikowska says, “found another way of getting the sugar—because it’s all about the sugar—by associating with an organism that can do photosynthesis.” More often than not, that organism is a type of green algae, but it can also be a photosynthetic bacterium (cyanobacteria, also called blue-green algae). It is still unclear how the mycobiont finds the matching photobiont if both partners are not dispersed together. Maybe the fungal spores (very small fungal reproductive unit) “will just sit and wait” until the right photobiont partner comes along. (How romantic.) Some mycobionts are specialists that “can only associate with a few or a single partner—a ‘species’ of Nostoc [a cyanobacterium; we still don’t know how many species of symbiotic and free-living Nostoc are out there and how to recognize them], for example,” but many are generalists with more flexible preferences.
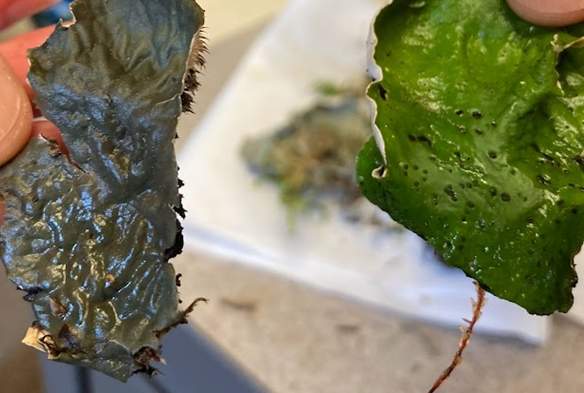
Lichens are classified based on their overall thallus shape. They can be foliose (leaf-like), fruticose (shrubby), or crustose (forming a crust on rocks or other surfaces). Lichens that grow on trees are epiphytic, while those that live on rocks are saxicolous; lichens that live on top of mosses are muscicolous, and ground-dwelling lichens are terricolous. Much of Miadlikowska’s research is on a group of cyanolichens (lichens with cyanobacteria partners) from the genus Peltigera. She works on the systematics and evolution of this group using morphology-, anatomy-, and chemistry-based methods and molecular phylogenetic tools. She is also part of a team exploring biodiversity, ecological rules, and biogeographical patterns in cryptic fungal communities associated with lichens and plants (endolichenic and endophytic fungi). She has been involved in multiple ongoing NSF-funded projects and also helping graduate students Ian, Carlos, Shannon, and Diego in their dissertation research. She spent last summer collecting lichens with Carlos and Shannon and collaborators in Alberta, Canada and Alaska. If you walk in the sub basement of the Bio Sciences building where Bio201 and Bio202 labs are located, check out the amazing photos of lichens (taken by Thomas Barlow, former Duke undergraduate) displayed along the walls! Notice Peltigera species, including some new to science, described by the Duke lichen team.
Lichens have value beyond the realm of research, too. “In traditional medicine, lichens have a lot of use,” Miadlikowska says. Aside from medicinal uses, they have also been used to dye fabric and kill wolves. Some are edible. Miadlikowska herself has eaten them several times. She had salad in China that was made with leafy lichens (the taste, she says, came mostly from soy sauce and rice vinegar, but “the texture was coming from the lichen.”). In Quebec, she drank tea made with native plants and lichens, and in Scandinavia, she tried candied Cetraria islandica lichen (she mostly tasted the sugar and a bit of bitterness, but once again, the lichen’s texture was apparent).
In today’s changing world, lichens have another use as well, as “bioindicators to monitor the quality of the air.” Most lichens can’t tolerate air pollution, which is why “in big cities… when you look at the trees, there are almost no lichens. The bark is just naked.” Lichen-covered trees, then, can be a very good sign, though the type of lichen matters, too. “The most sensitive lichens are the shrubby ones… like Usnea,” Miadlikowska says. Some lichens, on the other hand, “are able to survive in anthropogenic places, and they just take over.” Even on “artificial substrates like concrete, you often see lichens.” Along with being very sensitive to poor air quality, lichens also accumulate pollutants, which makes them useful for monitoring deposition of metals and radioactive materials in the environment.

LaGreca, like Miadlikoska, is a lichenologist. His research primarily concerns systematics, evolution and chemistry of the genus Ramalina. He’s particularly interested in “species-level relationships.” While he specializes in lichens now, LaGreca was a botany major in college. He’d always been interested in plants, in part because they’re so different from animals—a whole different “way of being,” as he puts it. He used to take himself on botany walks in high school, and he never lost his passion for learning the names of different species. “Everything has a name,” he says. “Everything out there has a name.” Those names aren’t always well-known. “Some people are plant-blind, as they call it…. They don’t know maples from oaks.” In college he also became interested in other organisms traditionally studied by botanists—like fungi. When he took a class on fungi, he became intrigued by lichens he saw on field trips. His professor was more interested in mushrooms, but LaGreca wanted to learn more, so he specialized in lichens during grad school at Duke, and now lichens are central to his job. He researches them, offers help with identification to other scientists, and is the collections manager for the lichens in the W.L. and C.F. Culberson Lichen Herbarium—all 160,000 of them.
The Duke Herbarium was founded in 1921 by Dr. Hugo Blomquist. It contains more than 825,000 specimens of vascular and nonvascular plants, algae, fungi, and, of course, lichens. Some of those specimens are “type” specimens, meaning they represent species new to science. A type specimen essentially becomes the prototype for its species and “the ultimate arbiter of whether something is species X or not.” But how are lichens identified, anyway?
Lichenologists can consider morphology, habitat, and other traits, but thanks to Dr. Chicita Culberson, who was a chemist and adjunct professor at Duke before her retirement, they have another crucial tool available as well. Culbertson created a game-changing technique to identify lichens using their chemicals, or metabolites, which are often species-specific and thus diagnostic for identification purposes. That technique, still used over fifty years later, is a form of thin-layer chromatography. The process, as LaGreca explains, involves putting extracts from lichen specimens—both the specimens you’re trying to identify and “controls,” or known samples of probable species matches—on silica-backed glass plates. The plates are then immersed in solvents, and the chemicals in the lichens travel up the paper. After the plates have dried, you can look at them under UV light to see if any spots are fluorescing. Then you spray the plates with acid and “bake it for a couple hours.” By the end of the process, the spots of lichen chemicals should be visible even without UV light. If a lichen sample has traveled the same distance up the paper as the control specimen, and if it has a similar color, it’s a match. If not, you can repeat the process with other possible matches until you establish your specimen’s chemistry and, from there, its identity. Culberson’s method helped standardize lichen identification. Her husband also worked with lichens and was a director of the Duke Gardens.

LaGreca shows me a workroom devoted to organisms that are cryptogamic, a word meaning “hidden gametes, or hidden sex.” It’s a catch-all term for non-flowering organisms that “zoologists didn’t want to study,” like non-flowering plants, algae, and fungi. It’s here that new lichen samples are processed. The walls of the workroom are adorned with brightly colored lichen posters, plus an ominous sign warning that “Unattended children will be given an espresso and a free puppy.” Tucked away on a shelf, hiding between binders of official-looking documents, is a thin science fiction novel called “Trouble with Lichen” by John Wyndham.
The Culberson Lichen Herbarium itself is a large room lined with rows of cabinets filled with stacks upon stacks of folders and boxes of meticulously organized lichen samples. A few shelves are devoted to lichen-themed books with titles like Lichens De France and Natural History of the Danish Lichens.
Each lichen specimen is stored in an archival (acid-free) paper packet, with a label that says who collected it, where, and on what date. (“They’re very forgiving,” says LaGreca. “You can put them in a paper bag in the field, and then prepare the specimen and its label years later.”) Each voucher is “a record of a particular species growing in a particular place at a particular time.” Information about each specimen is also uploaded to an online database, which makes Duke’s collection widely accessible. Sometimes, scientists from other institutions find themselves in need of physical specimens. They’re in luck, because Duke’s lichen collection is “like a library.” The herbarium fields loan requests and trades samples with herbaria at museums and universities across the globe. (“It’s kind of like exchanging Christmas presents,” says LaGreca. “The herbarium community is a very generous community.”)
Meticulous records of species, whether in databases of lichens or birds or “pickled fish,” are invaluable. They’re useful for investigating trends over time, like tracking the spread of invasive species or changes in species’ geographic distributions due to climate change. For example, some lichen species that were historically recorded on high peaks in North Carolina and elsewhere are “no longer there” thanks to global warming—mountain summits aren’t as cold as they used to be. Similarly, Henry David Thoreau collected flowering plants at Walden Pond more than 150 years ago, and his samples are still providing valuable information. By comparing them to present-day plants in the same location, scientists can see that flowering times have shifted earlier due to global warming. So why does Duke have tens of thousands of dried lichen samples? “It comes down to the reproducibility of science,” LaGreca says. “A big part of the scientific method is being able to reproduce another researcher’s results by following their methodology. By depositing voucher specimens generated from research projects in herbaria like ours, future workers can verify the results” of such research projects. For example, scientists at other institutions will sometimes borrow Duke’s herbarium specimens to verify that “the species identification is what the label says it is.” Online databases and physical species collections like the herbarium at Duke aren’t just useful for scientists today. They’re preserving data that will still be valuable hundreds of years from now.