In 2011, Dr. Jennifer Doudna began studying an enzyme called Cas9. Little did she know, in 2020 she would go on to win the Nobel Prize in Chemistry along with Emmanuelle Charpentier for discovering the powerful gene-editing tool, CRISPR-Cas9. Today, Doudna is a decorated researcher, the Li Ka Shing Chancellors Chair, a Professor in the Department of Chemistry and Molecular as well as Cell Biology at the University of California Berkeley, and the founder of the Innovative Genomics Institute.
Doudna was also this year’s speaker for the MEDx Distinguished Lecture in October where she delivered presented on “CRISPR: Rewriting DNA and the Future of Humanity.”
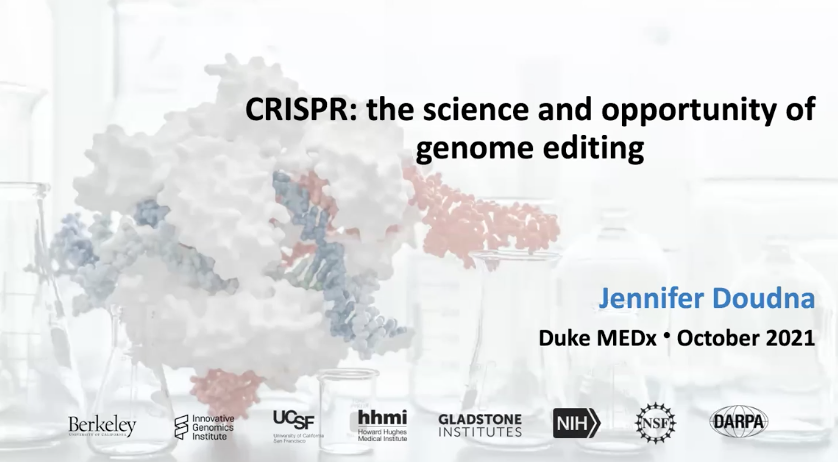
“CRISPR is a system that originated in bacteria as an adaptive immune system” Doudna explained.

When bacterial cells are infected by viruses those viruses inject their genetic material into the cell. This discovery, a couple decades ago, was the first indication that there may be ways to apply bacteria’s ability to acquire genetic information from viruses.
CRISPR itself was discovered in 1987 and stands for “Clustered Regularly Interspaced Short Palindromic Repeats.” Doudna was initially studying RNA when she discovered Cas-9, a bacterial RNA-guided endonuclease and one of the enzymes produced by the CRISPR system. In 2012, Doudna and her colleagues found that Cas9 used base pairing to locate and splice target DNAs when combined with a guide RNA.
Essentially, they designed guide RNA to target specific cells. If those cells had a CRISPR system encoded in their genome, the cell is able to make an RNA copy of the CRISPR locus. Those RNA molecules are then processed into units that each include a sequence derived from a virus and then assemble with proteins. This RNA protein then looks for DNA sequences that match the sequence in the RNA guide. Once a match occurs, Cas9 is able to bind to and cut the DNA, leading to the destruction of the viral genome. The cutting of DNA then triggers DNA repair allowing gene editing to occur.
“This system has been harnessed as a technology for genome editing because of the ability of these proteins, these CRISPR Cas-p proteins, to be programmed by RNA molecules to cut any desired DNA sequence,” Doudna said.

While continuing to conduct research, Doudna has also been focused on applying CRISPR in agriculture and medicine. For agriculture, researchers are looking to make changes to the genomes of plants in order to improve drought resistance and crop protection.
CRISPR-cas9 is also being applied in many clinical settings. In fact, when the COVID-19 pandemic hit, Doudna along with several colleagues organized a five-lab consortium including the labs of Dan Fletcher, Patrick Hsu, Melanie Ott, and David Savage. The focus was on developing the Cas13 system to detect COVID-19. Cas13 is a class of proteins, that are RNA guided, RNA targeting, CRISPR enzymes. This research was initially done by one of Doudna’s former graduate students, Alexandra East-Seletsky. They discovered that if the reporter RNA is is paired with enzymes that have a quenched fluorophore pair on the ends, when the target is activated, the reporter is cleaved and a fluorescent signal is released.
One study out of the Melanie Ott group demonstrated that Cas13 can be used to detect viral RNA. They are hoping to apply this as a point-of-care diagnostic by using a detector as well as a microfluidic chip which would allow for the conduction of these chemical reactions in much smaller volumes that can then be read out by a laser. Currently, the detection limit is similar to what one can get with a PCR reaction however it is significantly easier to run.

“And this is again, not fantasy, we’ve actually had just fabricated devices that will be sitting on a benchtop, and are able to use fabricated chips that will allow us to run the Cas13 chemistry with either nasal swab samples or saliva samples for detection of the virus,” Doudna added.
Another exciting development is the use of genome editing in somatic cells. This involves making changes in the cells of an individual as opposed to the germline. One example is sickle cell disease which is caused by a single base pair defect in a gene. Soon, clinicians will be able to target and correct this defect at the source of the mutation alleviating people from this devastating illness. Currently, there are multiple ongoing clinical trials including one at the Innovative Genomics Institute run by Doudna. In fact, one patient, Victoria Gray, has already been treated for her sickle cell disease using CRISPR.

Meredith Rizzo/NPR
“The results of these trials are incredibly exciting and encouraging to all of us in the field, with the knowledge that this technology is being deployed to have a positive impact on patient’s lives,” Doudna said.
Another important advancement was made last summer involving the use of CRISPR-based therapy to treat ATR, a rare genetic disease that primarily affects the liver. This is also the first time CRISPR molecules will be delivered in vivo.
In just 10 years CRISPR-cas9 has gone from an exciting discovery to being applied in several medical and agricultural settings.
“This powerful technology enables scientists to change DNA with precision only dreamed of a few years ago,” said MEDx director Geoffrey Ginsburg, a Professor of Medicine at Duke. “Labs worldwide have redirected the course of research programs to incorporate this new tool, creating a CRISPR revolution with huge implications across biology and medicine.”
Examples of further CRISPR-Cas9 research can also be found in the Charles Gersbach lab here at Duke.
